Introduction
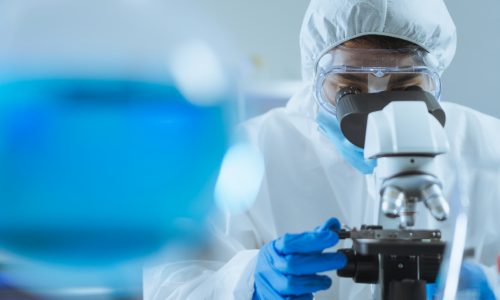
Hemocompatibility is a crucial property of medical device biocompatibility, specifically for surfaces that come in contact with blood. Blood is a complex biochemical system, responsible for functions such as the transport of oxygen, elimination of invading pathogens, and restoration of damaged tissues.
As a result, any device that encounters the circulatory system requires hemocompatibility testing to ensure that it is biocompatible.
Hemocompatibility testing
Hemocompatible testing is done to limit various adverse reactions such as coagulation, hemolysis, inflammation, or platelet activation. ISO 10993-4 gives international guidance on the selection of biocompatibility standardized test methods, where medical device manufacturers can evaluate isolated parameters through proven standardized test methods relevant to their device. Devices/materials are subjected to in vitro exposure of blood through static or dynamic means and examined for biomarkers as suggested by ISO 10993-4.
One of the challenges with uniform hemocompatibility testing is the high donor to donor variability for blood parameters being analyzed. Due to this, hemocompatibility testing is conducted as a comparative test. Device manufacturers need to simultaneous test to marketed comparator devices with known thrombogenicity profiles as recommended by the FDA, and test to validated controls, comparing to control samples to limit variability among blood parameters from different donors.
Static Testing
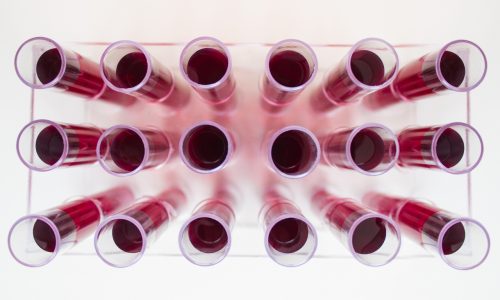
Static testing is a valuable tool to examine device hemocompatibility, where manufacturers can examine specific biomarkers of blood when in contact with a device material under controlled unagitated conditions. This involves exposing a test material or device to blood cell suspension in stationary conditions, where samples are incubated, monitored, and analyzed after set time points. This allows researchers a controlled, time and cost-effective evaluation of specific biomarkers as an initial screening step before dynamic testing is conducted. Static hemocompatibility is less sensitive than dynamic testing as it does not experience the same flow and surface interactions as dynamic testing, however, can be used to get data on surface and material hemocompatibility.
Dynamic testing
Rockers and Rotators
Dynamic testing assesses the interaction between blood and medical devices under physiological flow conditions, allowing manufacturers to mimic realistic conditions in vitro without the cost and ethical considerations of animal testing. A form of dynamic testing simulating the dynamic environment of blood flow in vitro is through use of a rocker or rotator. A rocker plate consists of a flat plate that rocks or tilts back and forth, while a rotator centrifugally agitates samples mimicking the mechanical and centrifugal forces experienced by blood when flowing through vessels or interacting with artificial surfaces within the body. Both devices play a role in hemocompatibility testing by providing a dynamic environment to study the effects of mechanical forces on blood-material interactions, aiding in the development of safer and more compatible medical devices.
Chandler Loop
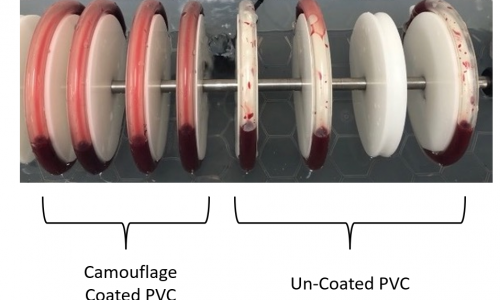
Another method of dynamically examining the effects of artificial surfaces on the activation of various cascade responses of the human hemostatic system is done through use of the Chandler-Loop model. This model consists of a closed-loop system where blood is circulated continuously through a test chamber containing the device/material being evaluated.
The picture above illustrates Smart Reactors Camouflage™ coated tubing (left) tested against uncoated tubing (right) after being subjected to human blood in a chandler loop for 60 minutes. Camouflage™ allows for uniform blood adhesion to the inner wall through enhancing device wettability, while the control group (uncoated PVC) leaves irregular patches of blood along the surface.
Blood is first withdrawn from a donor and anticoagulated to prevent clotting. Blood Samples are typically acquired through the form of human donors or animal reagent kits. The necessary blood volume is device dependent; however, ISO 10993-12 can provide guidance. Human samples, typically lightly heparinized to keep the medium reactive, should be used as soon as possible post-extraction.
The blood is then pumped through the test chamber, where it interacts with the surface of the biomaterial or medical device. The blood is continuously recirculated through the loop, allowing for prolonged exposure of the material to blood components. The chandler loop model allows for the most comprehensive in vitro analysis of blood-material interactions, subjecting devices to cyclical and bilateral forces simulating physiological circulation.
Small diameter devices such as stents and flow diverters present unique challenges in testing due to their intricate design and intended application sites within the body. A modified chandler loop model can be leveraged, where a heated water bath and pump simulate physiological fluid flow conditions within blood vessels.
Biomarkers and how they are evaluated
ISO 10993-4 outlines procedures for evaluating the potential adverse effects of medical devices when in contact with blood, including both systemic effects, such as coagulation disorders along with local effects, such as thrombosis or hemorrhage formation at the device site.
The type of tests and biomarkers required for a given medical device depend on the blood contact category of the device or material. This can be broken down into two categories, where external communicating devices indirectly interact with the blood path, while internal communicating devices directly are implanted or come into direct contact with circulating blood. The following biomarkers are outlined below, conforming to ISO 10993-4.
Coagulation
Coagulation is measured through plasma concentrations of Thrombin-Antithrombin complex (TAT), examined through enzyme-linked immunosorbent assays (ELISA). When a device encounters blood, internal clotting processes are triggered encompassing factors XII, XI, and ultimately factor X, which regulates the conversion of prothrombin. As thrombin forms, it splits off a fragment of prothrombin (F 1+2). The resulting thrombin is deactivated by binding with antithrombin, forming what is known as the TAT complex. Immunochemical measurement of TAT in plasma serves as a highly effective marker for detecting activated clotting. Through ELISA measurements, the advancement of blood samples toward coagulation serves as an indication of fibrin network formation, allowing device manufactures to examine this univariate property.
Platelets
When blood encounters artificial surfaces, the activation and modification of platelets are triggered, resulting in a decline in their functionality. This happens first through shape change, through the formation of pseudopodia. Subsequently, platelets adhere to the device surfaces, then begin to aggregate, leading to a decrease in platelet count. This decrease serves as a indicator of the compatibility of blood-contacting devices, which is examined through hematology analysis. Post-aggregation, the activation of platelets is examined through the release of platelet factors from the α-granules, with biomarkers β-Thrombo-globulin and Platelet Factor 4 which are examined using ELISA.
Hemolysis
When artificial surfaces encounter blood, high shear forces can disrupt, activate, and modify blood cells, resulting in decreased functionality of the cardiovascular system. If the membranes of RBCs are disrupted, hemoglobin is released and can be quantified and measured as free hemoglobin in plasma, a process known as hemolysis. This event leads to a reduction in red blood cell count and an increase in hemolysis, serving as an indicator for hemocompatibility. As a result, hemolysis is measured through spectrophotometry, specifically the cyanmethemoglobin method where the cyanide derivative of hemoglobin is measured through the addition of potassium cyanide and ferricyanide to blood.
Hematology
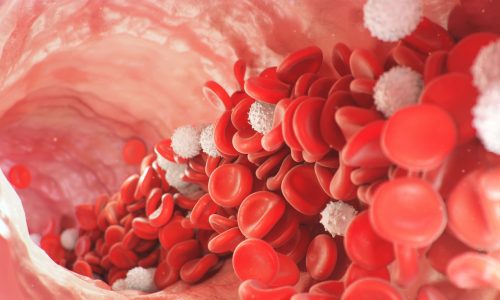
Hematology refers to the analysis of blood cells, specifically cell count in this case. When white blood cells (WBC) encounter artificial surfaces, they can adhere to them and engage in combat against perceived pathogens. This response results in a decrease in leukocyte (WBC) count, serving as an indicator of hemocompatibility.
Similarly, high shear forces cause the destruction of red blood cell (RBC) membranes, leading to a reduction in erythrocyte (RBC) count. WBC, RBC, and platelets serve as biomarkers for the blood environment after a device has interacted with the cardiovascular system. WBC and RBC are examined through use of hematology analyzers, specifically through hematocrit and hemoglobin measurements, respectively.
Partial Thromboplastin Time (PTT)
Partial Thromboplastin Time (PTT) assesses the time it takes for blood to form a clot, specifically on material-mediated impacts to the intrinsic coagulation pathway by introducing the test sample into platelet-poor citrated plasma. Subsequently, PTT reagent and calcium chloride solution are introduced, and the time until clot formation is observed and documented. Statistical analysis is then employed to compare clotting times with control samples.
Complement System
Activation of the complement system occurs when blood interacts with materials, serving as a defensive response against perceived pathogens. Inflammation and immune cells are recruited through anaphylatoxins C3a and C5a, which are released at activation. During the terminal stage, membrane attack complex (SC5b-9) is formed to emerge as a highly sensitive marker for cell lysis, thus holding significant importance in assessing the hemocompatibility of artificial devices. SC5b-9, C3a, and C5a not only indicate the degree of complement system engagement, but also aid in evaluating the immune response and level of coagulation triggered by the device’s surface interaction with blood components, which can be assessed using ELISAs.
Leukocyte Activation (Inflammation)
The activation of PMN-Elastase-α1-P1 serves as an indicator for an immune response mediated by leukocyte activation. PMN-elastase, otherwise known as neutrophil elastase, released by polymorphonuclear leukocytes (PMNs), play a role in the immune response, particularly in inflammation and tissue remodeling. When polymorphonuclear granulocytes become activated, they express membrane proteins like CD 11b/c and release myeloperoxidase and elastase from their granules. The unbound elastase forms complexes with α1-proteinase inhibitors. The concentration of this complex in plasma is measured using ELISA techniques, which can be utilized to gauge granulocyte activation during inflammatory responses.
Surface Analysis
Utilizing techniques such as scanning electron microscopy (SEM) or light microscopy allows for microscopic evaluation of surfaces, enabling the identification of challenge areas and the extent of adherence of fibrin, platelets, and blood cells to device surfaces. This allows researchers to detect or examine irregularities, design flaws, or roughness that can influence cell response. SEM in particular offers high-resolution imaging, revealing detailed surface morphology and the distribution of adherent blood components. This microscopic surface analysis aids in understanding the mechanisms underlying blood-material interactions, guiding the design and optimization of medical devices to enhance their hemocompatibility and ultimately improve patient outcomes.
In Vivo Studies
For blood contacting medical devices, device associated thrombosis evaluation in vivo may be required as outlined in ISO 10993-4. Depending on the device intended use, either anticoagulated or non-anticoagulated models in the venous or arterial environment of the animal are employed. The non-anticoagulated venous implant (NAVI) and anticoagulated implant (AVI) allow for catheter-type devices to be subjected to the veins of animals for up to 4 hours, where the amount of thrombus on the device surface is quantified. The canine femoral or jugular vein model is most commonly used, where a test material device is positioned up to 15cm in one vein and a control material or predicate device is placed in the contralateral site. The test requires two to three large animals, with alternating test and control implant locations to avoid bias.
Other large animal models can be used, such as the long-term heart lung model for ECMO applications, where cannulation is done through the jugular vein for venous drainage and the artery for arterial reinfusion. This allows testing on awake animals undergoing veno-arterial extracorporeal membrane oxygenation for days on end. Each in vivo model is device dependent, allowing device manufacturers to examine device specific parameters through simulated clinical models, examining biomarkers in vivo.
Benefits of Hemocompatible Coatings
Hemocompatible coatings on medical devices offer numerous benefits and enhance properties of medical devices, reducing the adverse effects in vitro discussed throughout this article. Many device materials with surfaces that are known to have poor hemocompatibility (e.g. Polypropylene (PP) and Polymethylpentene (PMP) Hollow Fiber Membranes) require coatings to improve surface performance when in contact with the patient’s blood stream. These coatings can help reduce thrombogenicity by minimizing platelet adhesion and clot formation, thereby lowering the risk of thromboembolism and device malfunction. Additionally, many can promote endothelization on the device surface.
The article on Hemocompatible coatings in the resource page on the website dives into the differences between leading industry surface coatings, such as Smart Reactors Camouflage™ coating, which will enhance the hemocompatibility, safety, efficacy, and longevity of the device surface, benefiting both patients & healthcare providers in the short, medium and longer term.
Share this post: on LinkedIn