Understanding Hemocompatibility
Medical Devices that contact blood can induce clot formation if they are not designed to be hemocompatible. The circulating blood recognizes foreign surfaces and initiates changes in the which results in thrombus (clot) formation. The presence of clots on device surfaces poses a substantial risk to patients and can foul devices affecting their performance. If thrombi are created which leave the surface of the device these can lead to emboli and can block blood vessels in distant organs and tissues leading to further patient harm. The level of risk associated with the performance of a device in blood must be understood and the surface must be designed to minimize the reactivity of the blood to the device.
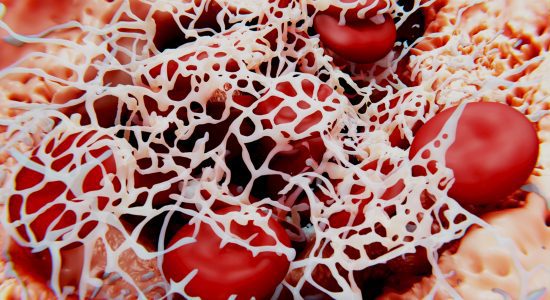
The risk of a device depends on where in the bloodstream it will be placed and for how long. For example, a neurovascular device (such as a flow diverter for aneurysm treatment) placed in an artery in the brain poses a risk to critical brain tissue if the device is not hemocompatible. Venous devices (such as peripheral venous stents or filters) can lead to pulmonary embolization of clot fragments if there is a reaction to the surface by venous blood. Perfusion Circuits containing Oxygenators pose substantial hemocompatibility challenges due to the large contact area and volume of blood flow through them. During the early stage of product design, careful consideration must be given to the risks associated with the potential of the clotting cascade. Product designers should be familiar with best practices in evaluating the performance of devices in blood, Including the molecular mechanisms that lead to thrombogenicity on the device surface.
Mechanisms of Blood Coagulation
In order to understand the risk of clot formation on the surface of a blood-contacting medical device one must first look at the mechanisms that take part in the formation of that clot. To put it in simple terms, when a biomaterial contacts blood it triggers a series of cellular and molecular reactions within the coagulation pathway. There are two different types of coagulation pathways known as the extrinsic and intrinsic pathways. The extrinsic pathway occurs when a blood vessel is damaged, whereas the intrinsic pathway is initiated when blood comes into contact with a foreign material such as a medical device. Both pathways, however, penultimately produce a molecule known as thrombin which is a key enzyme in the coagulation pathway and therefore in thrombus formation.
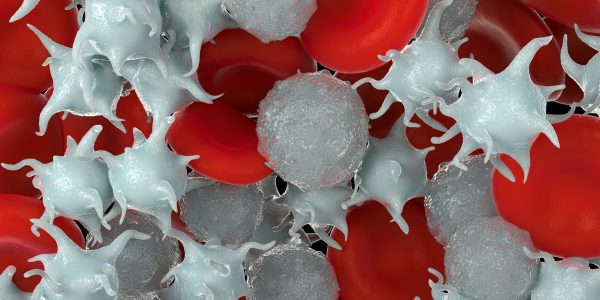
Thrombin cleaves fibrinogen, a soluble plasma protein, to form fibrin strands. These strands polymerize to form a meshwork that not only stabilizes the clot but also provides a scaffold for the entrapment of blood cells, platelets, and other plasma proteins.
Thrombin also amplifies the coagulation process by activating platelets and several other clotting factors. On contact with blood, plasma proteins instantaneously deposit onto the surface of the device. This is seen as the preliminary step in triggering clot activation. The order in which the proteins deposit onto the surface is dictated by
the Vroman effect which describes the process and order of protein adsorption to the surface. In the first instance, the lower molecular weight high mobility proteins such as albumin are adsorbed. These proteins are later replaced by the lower mobility higher molecular weight proteins such as fibrinogen.
Fibrinogen plays a vital role in the formation of fibrin strands which forms the basis of a blood clot but fibrinogen which is adsorbed onto the surface of a medical device allows the adherence of platelets. In this instance, the platelet undergoes a shape change and activation, and it has been shown that activated platelets contribute significantly to thrombotic complications. Activated platelets lead to both platelet aggregation as well as the release of other biomolecules involved in coagulation such as the previously discussed thrombin.
There are a variety of other blood cells that play a role in thrombus formation, for example, neutrophils and monocytes. Neutrophils are the immune system’s first response and are the basis of neutrophil extracellular traps (NETs). These are a network of extracellular fibers that bind pathogens, but they also play a role in thrombus formation. Similar to fibrin NETs provide a structure for the deposition of platelets and other biological substances which are conducive to thrombus.
Overall understanding the intrinsic pathway plays a crucial role in hemocompatibility and developing blood-contacting medical devices. Through a series of sequential reactions involving various clotting factors, the pathway culminates in the formation of a stable blood clot in response to contact with a biomaterial. As previously discussed, this could have detrimental effects on the patient’s health and could cause a series of problems.
Device Factors that influence hemocompatibility
In addition to the functional design of a medical device biomedical engineers must give consideration to the hemocompatibility. This is a complex and challenging interaction that is affected by blood flow dynamics, duration of use, the geometry of the device, the material and surface energy of the device, the total surface area and the surface roughness of the device.
All these parameters must be optimized to reduce the risk of a pro-coagulation reaction to the device in the body. Coatings, such as the Smart Reactors Camouflage™ coating demonstrates excellent hemocompatibility on devices that have a sound baseline design. The geometry of the device can lead to disruption of blood flow creating higher wall shear of blood against the surface of the device which is known to increase the activation of platelets and progression towards thrombus. Conversely, stagnant blood is also prone to clotting and “dead” zones with little to no flow are likely to induce pro-coagulant conditions.
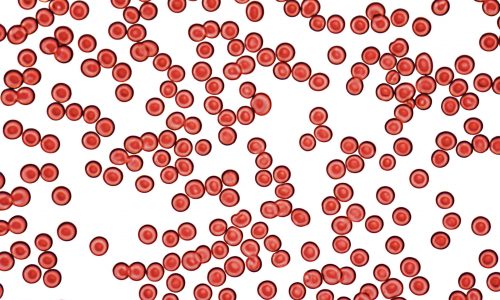
The surface chemistry of the device can lead to interactions with circulating proteins that can increase the attraction of proinflammatory and procoagulant proteins on the device’s surface, poor surface chemistry can also increase the rate of hemolysis and damage to red blood cells. In general, keeping the device surfaces smooth on a microscopic level is preferred and generates less procoagulant interaction with circulating platelets and blood proteins.
Evaluation of Hemocompatibility
The medical device design process needs to consider evaluating a device’s hemocompatibility at an early stage to ensure its ability to perform in blood is “designed in.” Various in-vitro and in-vivo tests have been developed that consider the complexity of the clotting cascade and the device’s surface/blood interactions.
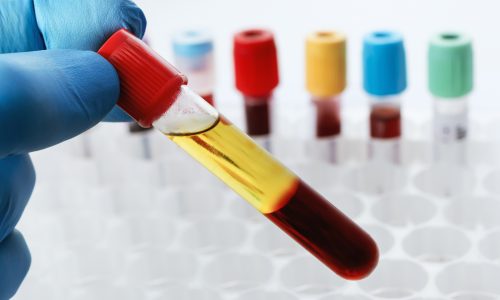
The most common in-vitro assays rely on either static or dynamic blood contact. In-vitro dynamic testing produces the most detailed laboratory testing for evaluating a device’s performance without the cost, complexity, and ethical considerations of animal testing.
This testing can also be performed on individual materials and device sub-systems at a time when the entire device may not be ready for in-vivo evaluation, allowing developers to get an early indicator of device performance.
The table below shows some of the common test methods that are used and which cover the performance of a device with respect to the different mechanisms of clot initiation.
Category | Test | Purpose | |
Coagulation | Measurement of Thrombin-Antithrombin (TAT) complex using ELISA measurement | The purpose of the TAT Elisa assay is to determine the progression of sample blood towards coagulation as an indicator of the formation of fibrin networks. | |
Hemolysis | The measurement of Free Hemoglobin by spectrophotometry | This measurement provides an indicator for how many erythrocytes are being lysed (freeing up hemoglobin). This tells how likely the device surface is to damage blood and lead to pro-coagulant constituents being released. | |
Hematology | Measurement of circulating cell counts (Platelets, White Blood Cells, and Red Blood Cells) using commercial hematology analyzers | Reduction in circulating cell counts suggest that cells have either adsorbed on the surface or otherwise been incorporated into a blood clot. | |
Platelet | Measurement of markers of platelet activation such as Beta-Thromboglobulin or Platelet Factor 4 using an ELISA Assay | The biological markers indicating platelet activation | |
Complement | Measurement of complement activation markers such as C3a, C5a and SC5b9 using an ELISA assay | The activation of the complement system indicates an innate immune response to the surface of the device which can lead to coagulation | |
Leukocyte Activation | Measurement of PMN Elastase using an ELISA Assay | The activation of PMN elastase indicates that there is an immune response mediated by leukocytes. | |
Surface analysis | Microscopic Surface evaluation using SEM or light microscopy | The visual appearance of surfaces, particularly under SEM, can identify challenge areas and adherence of fibrin, platelets, and blood cells | |
Improving Device Hemocompatibility with surface modification
Surface coatings are known to provide improvements in the performance of medical devices, particularly those with surfaces that are known to have poor hemocompatibility (e.g. Polypropylene (PP) and polymethylpentene (PMP) Hollow Fiber Membranes). Smart Reactors article on hemocompatible coatings will delve further into the chemistry of these technologies. In General, improving the wettability of surfaces will improve their hemocompatibility and their performance in blood.
Conclusion
A device with poor hemocompatibility presents a risk to patient health that is application-specific. Engineers and scientists must understand the performance of their device in contact with the patient’s bloodstream and take steps to minimize the risk of thrombogenicity. The device materials, anatomical positioning, surface texture, and duration of use all contribute to the performance of the device. Standard test methods are designed that cover the many intricate pathways of the clotting cascade to provide wide assurance that the device surface is not going to pose a risk to patients. Surface coatings such as Smart Reactors Camouflage™ will enhance the hemocompatibility of the device surface and support medical device manufacturers to deliver products that are safer and more effective for patients.
Share this post: on LinkedIn